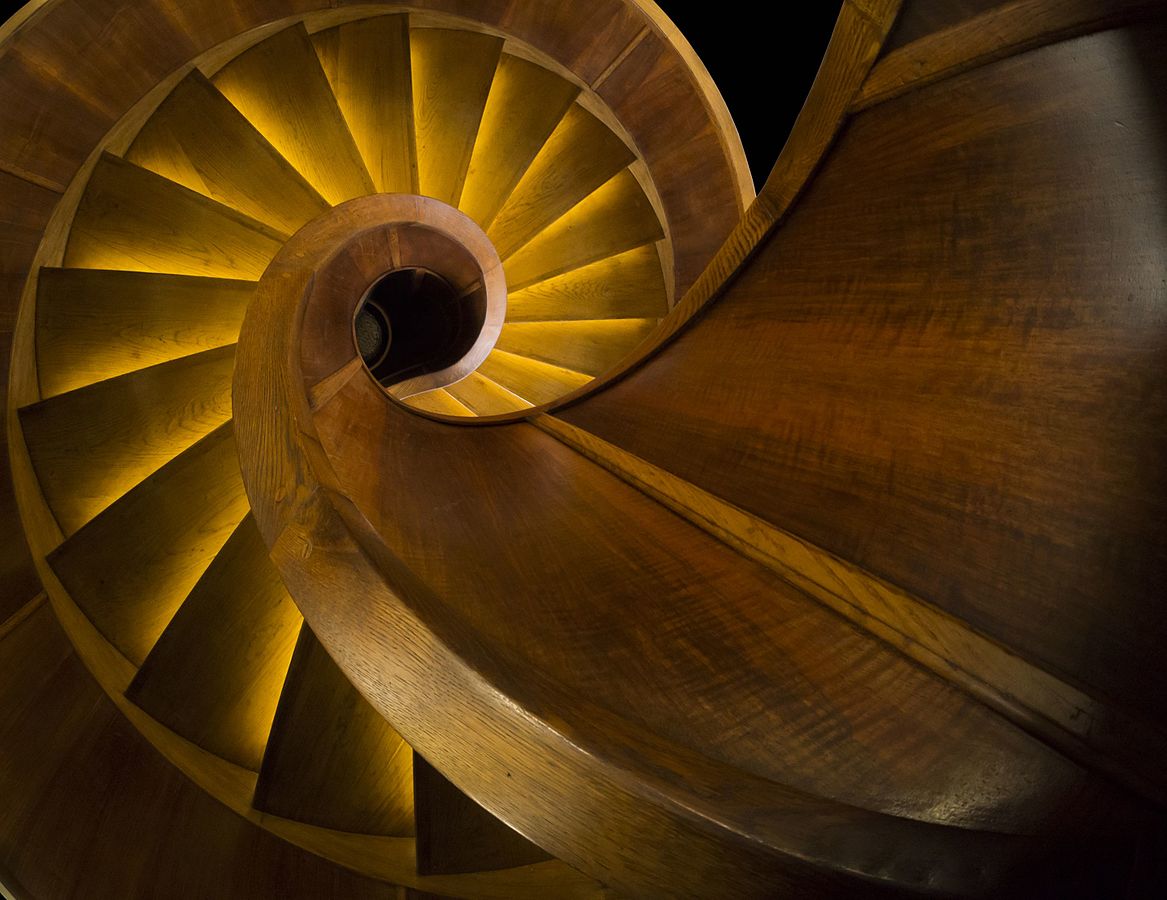
A few years ago, I had observed after hearing a talk by Benjamin Rin that the principle of first-order transfinite recursion for set well-orders is equivalent to the replacement axiom over Zermelo set theory, and thus we may take transfinite recursion as a fundamental set-theoretic principle, one which yields full ZFC when added to Zermelo’s weaker theory (plus foundation).
In later work, Victoria Gitman and I happened to prove that the principle of elementary transfinite recursion ETR, which allows for first-order class recursions along proper class well-orders (not necessarily set-like) is equivalent to the principle of determinacy for clopen class games [1]. Thus, once again, a strong recursion principle exhibited robustness as a fundamental set-theoretic principle.
The theme continued in recent joint work on the class forcing theorem, in which Victoria Gitman, myself, Peter Holy, Philipp Schlicht and Kameryn Williams [2] proved that the principle $\text{ETR}_{\text{Ord}}$, which allows for first-order class recursions of length $\text{Ord}$, is equivalent to twelve natural set-theoretic principles, including the existence of forcing relations for class forcing notions, the existence of Boolean completions for class partial orders, the existence of various kinds of truth predicates for infinitary logics, the existence of $\text{Ord}$-iterated truth predicates, and to the principle of determinacy for clopen class games of rank at most $\text{Ord}+1$.
A few days ago, a MathOverflow question of Alec Rhea’s — Is there a stronger form of recursion? — led me to notice that one naturally gains additional strength by pushing the recursion principles further into second-order set theory.
So let me introduce the second-order recursion principle STR and make the comparatively simple observation that over Gödel-Bernays GBC set theory this is equivalent to Kelley-Morse set theory KM. Thus, we may take this kind of recursion as a fundamental set-theoretic principle.
Definition. In the context of second-order set theory, the principle of second-order transfinite recursion, denoted STR, asserts of any formula $\varphi$ in the second-order language of set theory, that if $\Gamma=\langle I,\leq_\Gamma\rangle$ is any class well-order and $Z$ is any class parameter, then there is a class $S\subset I\times V$ that is a solution of the recursion, in the sense that
$$S_i=\{\ x\ \mid\ \varphi(x,S\upharpoonright i,Z)\ \}$$
for every $i\in I$, where where $S_i=\{\ x\ \mid\ (i,x)\in S\ \}$ is the section on coordinate $i$ and where $S\upharpoonright i=\{\ (j,x)\in S\ \mid\ j<_\Gamma i\ \}$ is the part of the solution at stages below $i$ with respect to $\Gamma$.
Theorem. The principle of second-order transfinite recursion STR is equivalent over GBC to the second-order comprehension principle. In other words, GBC+STR is equivalent to KM.
Proof. Kelley-Morse set theory proves that every second-order recursion has a solution in the same way that ZFC proves that every set-length well-ordered recursion has a solution. Namely, we simply consider the classes which are partial solutions to the recursion, in that they obey the recursive requirement, but possibly only on an initial segment of the well-order $\Gamma$. We may easily show by induction that any two such partial solutions agree on their common domain (this uses second-order comprehension in order to find the least point of disagreement, if any), and we can show that any given partial solution, if not already a full solution, can be extended to a partial solution on a strictly longer initial segment. Finally, we show that the common values of all partial solutions is therefore a solution of the recursion. This final step uses second-order comprehension in order to define what the common values are for the partial solutions to the recursion.
Conversely, the principle of second-order transfinite recursion clearly implies the second-order comprehension axiom, by considering recursions of length one. For any second-order assertion $\varphi$ and class parameter $Z$, we may deduce that $\{x\mid \varphi(x,Z)\}$ is a class, and so the second-order class comprehension principle holds. $\Box$
It is natural to consider various fragments of STR, such as $\Sigma^1_n\text{-}\text{TR}_\Gamma$, which is the assertion that every $\Sigma^1_n$-formula $\varphi$ admits a solution for recursions of length $\Gamma$. Such principles are provable in proper fragments of KM, since for a given level of complexity, we only need a corresponding fragment of comprehension to undertake the proof that the recursion has a solution. The full STR asserts $\Sigma^1_\omega\text{-}\text{TR}$, allowing any length. The theorem shows that STR is equivalent to recursions of length $1$, since once you get the second-order comprehension principle, then you get solutions for recursions of any length. Thus, with second-order transfinite recursion, a little goes a long way. Perhaps it is more natural to think of transfinite recursion in this context not as axiomatizing KM, since it clearly implies second-order comprehension straight away, but rather as an apparent strengthening of KM that is actually provable in KM. This contrasts with the first-order situation of ETR with respect to GBC, where GBC+ETR does make a proper strengthening of GBC.
- [bibtex key=”GitmanHamkins2016:OpenDeterminacyForClassGames”]
- [bibtex key=”GitmanHamkinsHolySchlichtWilliams:The-exact-strength-of-the-class-forcing-theorem”]