Welcome to the Oxford Set Theory Seminar.
We focus on all aspects of set theory and the philosophy of set theory. Topics will include forcing, large cardinals, models of set theory, set theory as a foundation, set-theoretic potentialism, cardinal characteristics of the continuum, second-order set theory and class theory, and much more.
Technical topics are completely fine. Speakers are encouraged to pick set-theoretic topics having some philosophical angle or aspect, although it is expected that this might sometimes be a background consideration, while at other times it will be a primary focus.
The seminar will last 60-90 minutes, and are generally held on Wednesdays 4:00 – 5:30 UK time. Speakers are requested to prepare a one hour talk, and we expect a lively discussion with questions.
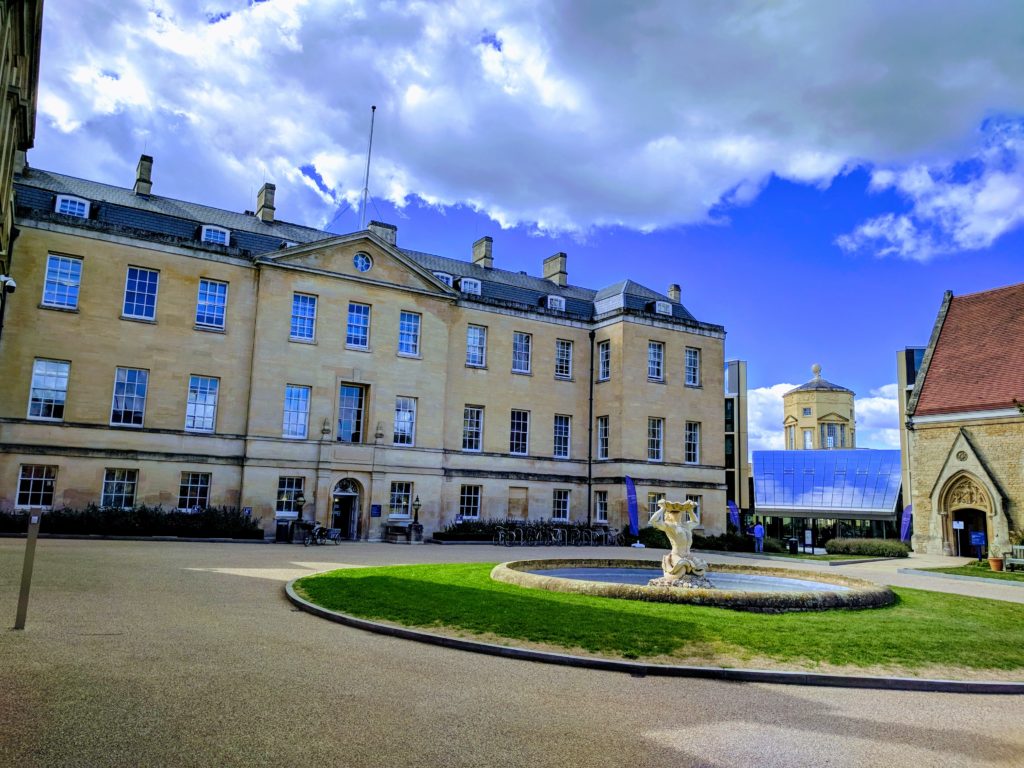
Trinity Term 2021
The seminar this term is again held jointly with the University of Bristol, organized by myself, Samuel Adam-Day, and Philip Welch.
For the Zoom access code, contact Samuel Adam-Day at me@samadamday.com.
19 May 2021 4:30 pm UK
Samuel Adam-Day
University of Oxford
The continuous gradability of the cut-point orders of $\newcommand\R{\mathbb{R}}\R$-trees
Abstract. An $\R$-tree is a metric space tree in which every point can be branching. Favre and Jonsson posed the following problem in 2004: can the class of orders underlying $\R$-trees be characterised by the fact that every branch is order-isomorphic to a real interval? In the first part of the talk, I answer this question in the negative: there is a branchwise-real tree order which is not continuously gradable. In the second part, I show that a branchwise-real tree order is continuously gradable if and only if every embedded well-stratified (i.e. set-theoretic) tree is $\R$-gradable. This tighter link with set theory is put to work in the third part answering a number of refinements of the main question, yielding several independence results.
26 May 2021 4:30 pm BST
Sandra Müller
TU Wien
The strength of determinacy when all sets are universally Baire
Abstract. The large cardinal strength of the Axiom of Determinacy when enhanced with the hypothesis that all sets of reals are universally Baire is known to be much stronger than the Axiom of Determinacy itself. In fact, Sargsyan conjectured it to be as strong as the existence of a cardinal that is both a limit of Woodin cardinals and a limit of strong cardinals. Larson, Sargsyan and Wilson showed that this would be optimal via a generalization of Woodin’s derived model construction. We will discuss a new translation procedure for hybrid mice extending work of Steel, Zhu and Sargsyan and use this to prove Sargsyan’s conjecture.
1 June 2021 4:30 pm BST
Christopher Turner
Bristol
Forcing Axioms and Name Principles
Abstract. Forcing axioms are a well-known way of expressing the concept ”there are filters in V which are close to being generic”. Name principles are another expression of this concept. A name principle says: ”Let $\sigma$ be any sufficiently nice name which is forced to have some property. Then there is a filter $g\in V$ such that $\sigma^g$ has that property.” Name principles have often been used on an ad-hoc basis in proofs, but have not been studied much as axioms in their own right. In this talk, I will present some of the connections between different name principles, and between name principles and forcing axioms. This is based on joint work with Philipp Schlicht.
16 June 2021 4:30 pm BST
Joan Bagaria
Barcelona
Some recent results on Structural Reflection
Abstract. The general Structural Reflection (SR) principle asserts that for every definable, in the first-order language of set theory, possibly with parameters, class $\mathcal{C}$ of relational structures of the same type there exists an ordinal $\alpha$ that reflects $\mathcal{C}$, i.e., for every $A$ in $\mathcal{C}$ there exists $B$ in $\mathcal{C}\cap V_\alpha$ and an elementary embedding from $B$ into $A$. In this form, SR is equivalent to Vopenka’s Principle (VP). In my talk I will present some different natural variants of SR which are equivalent to the existence some well-known large cardinals weaker than VP. I will also consider some forms of SR, reminiscent of Chang’s Conjecture, which imply the existence of large cardinal principles stronger than VP, at the level of rank-into-rank embeddings and beyond. The latter is a joint work with Philipp Lücke.
Hilary Term 2021
The seminar this term is again held jointly with the University of Bristol, organized by myself, Samuel Adam-Day, and Philip Welch.
For the Zoom access code, contact Samuel Adam-Day at me@samadamday.com.
Talks are held Wednesdays 4:00 – 5:30 pm UK time.
20 January 2021 4pm UK
Dima Sinapova
University of Illinois at Chicago
Iteration, reflection, and singular cardinals
Abstract. Two classical results of Magidor are:
(1) from large cardinals it is consistent to have reflection at $\aleph_{\omega+1}$, and
(2) from large cardinals it is consistent to have the failure of SCH at $\aleph_\omega$.
These principles are at odds with each other. The former is a compactness type principle. (Compactness is the phenomenon where if a certain property holds for every smaller substructure of an object, then it holds for the entire object.) In contrast, failure of SCH is an instance of incompactness. The natural question is whether we can have both of these simultaneously. We show the answer is yes.
We describe a Prikry style iteration, and use it to force stationary reflection in the presence of not SCH. Then we obtain this situation at $\aleph_\omega$. This is joint work with Alejandro Poveda and Assaf Rinot.
3 February 2021 4pm UK
Spencer Unger
University of Toronto
Stationary reflection at successors of singular cardinals
We survey some recent progress in understanding stationary reflection at successors of singular cardinals and its influence on cardinal arithmetic:
1) In joint work with Yair Hayut, we reduced the consistency strength of stationary reflection at aleph_{omega+1} to an assumption weaker than kappa is kappa+ supercompact.
2) In joint work with Yair Hayut and Omer Ben-Neria, we prove that from large cardinals it is consistent that there is a singular cardinal nu of uncountable cofinality where the singular cardinal hypothesis fails at nu and every collection of fewer than cf(nu) stationary subsets of nu+ reflects at a common point.
The statement in the second theorem was not previously known to be consistent. These results make use of analysis of Prikry generic objects over iterated ultrapowers.
24 March 2021 4pm UK
Andrew Marks
UCLA
The decomposability conjecture
Abstract. We characterize which Borel functions are decomposable into a countable union of functions which are piecewise continuous on $\Pi^0_n$ domains, assuming projective determinacy. One ingredient of our proof is a new characterization of what Borel sets are $\Sigma^0_n$ complete. Another important ingredient is a theorem of Harrington that there is no projective sequence of length $\omega_1$ of distinct Borel sets of bounded rank, assuming projective determinacy. This is joint work with Adam Day.
10 March 2021 4pm UK
Peter Koellner
Harvard University
Minimal Models and $\beta$ Categoricity
Abstract. Let us say that a theory $T$ in the language of set theory is $\beta$-consistent at $\alpha$ if there is a transitive model of $T$ of height $\alpha$, and let us say that it is $\beta$-categorical at $\alpha$ iff there is at most one transitive model of $T$ of height $\alpha$. Let us also assume, for ease of formulation, that there are arbitrarily large $\alpha$ such that $\newcommand\ZFC{\text{ZFC}}\ZFC$ is $\beta$-consistent at $\alpha$.
The sentence $\newcommand\VEL{V=L}\VEL$ has the feature that $\ZFC+\VEL$ is $\beta$-categorical at $\alpha$, for every $\alpha$. If we assume in addition that $\ZFC+\VEL$ is $\beta$-consistent at $\alpha$, then the uniquely determined model is $L_\alpha$, and the minimal such model, $L_{\alpha_0}$, is model of determined by the $\beta$-categorical theory $\ZFC+\VEL+M$, where $M$ is the statement “There does not exist a transitive model of $\ZFC$.”
It is natural to ask whether $\VEL$ is the only sentence that can be $\beta$-categorical at $\alpha$; that is, whether, there can be a sentence $\phi$ such that $\ZFC+\phi$ is $\beta$-categorical at $\alpha$, $\beta$-consistent at $\alpha$, and where the unique model is not $L_\alpha$. In the early 1970s Harvey Friedman proved a partial result in this direction. For a given ordinal $\alpha$, let $n(\alpha)$ be the next admissible ordinal above $\alpha$, and, for the purposes of this discussion, let us say that an ordinal $\alpha$ is minimal iff a bounded subset of $\alpha$ appears in $L_{n(\alpha)}\setminus L_\alpha$. [Note that $\alpha_0$ is minimal (indeed a new subset of $\omega$ appears as soon as possible, namely, in a $\Sigma_1$-definable manner over $L_{\alpha_0+1}$) and an ordinal $\alpha$ is non-minimal iff $L_{n(\alpha)}$ satisfies that $\alpha$ is a cardinal.] Friedman showed that for all $\alpha$ which are non-minimal, $\VEL$ is the only sentence that is $\beta$-categorical at $\alpha$. The question of whether this is also true for $\alpha$ which are minimal has remained open.
In this talk I will describe some joint work with Hugh Woodin that bears on this question. In general, when approaching a “lightface” question (such as the one under consideration) it is easier to first address the “boldface” analogue of the question by shifting from the context of $L$ to the context of $L[x]$, where $x$ is a real. In this new setting everything is relativized to the real $x$: For an ordinal $\alpha$, we let $n_x(\alpha)$ be the first $x$-admissible ordinal above $\alpha$, and we say that $\alpha$ is $x$-minimal iff a bounded subset of $\alpha$ appears in $L_{n_x(\alpha)}[x]\setminus L_{\alpha}[x]$.
Theorem. Assume $M_1^\#$ exists and is fully iterable. There
is a sentence $\phi$ in the language of set theory with two
additional constants, c and d, such that for a Turing cone
of $x$, interpreting c by $x$, for all $\alpha$
- if $L_\alpha[x]\models\ZFC$ then there is an interpretation of d by something in $L_\alpha[x]$ such that there is a $\beta$-model of $\ZFC+\phi$ of height $\alpha$ and not equal to $L_\alpha[x]$, and
- if, in addition, $\alpha$ is $x$-minimal, then there is a unique $\beta$-model of $\ZFC+\phi$ of height $\alpha$ and not equal to $L_\alpha[x]$.
The sentence $\phi$ asserts the existence of an object which is external to $L_\alpha[x]$ and which, in the case where $\alpha$ is minimal, is canonical. The object is a branch $b$ through a certain tree in $L_\alpha[x]$, and the construction uses techniques from the HOD analysis of models of determinacy.
In this talk I will sketch the proof, describe some additional features of the singleton, and say a few words about why the lightface version looks difficult.
Michaelmas Term 2020
This term, we are coordinating the seminar in collaboration with Bristol, and so let me announce the joint meetings of the Oxford Set Theory Seminar and the Bristol Logic and Set Theory seminar. Organized by myself, Samuel Adam-Day, and Philip Welch.
For the Zoom access code (which is the same as last term), contact Samuel Adam-Day me@samadamday.com.
Talks are held on Wednesdays 4:00 – 5:30 UK time.
21 October 2020 4 pm UK
Andreas Blass
University of Michigan
Ultrafilters on omega versus forcing
Abstract. I plan to survey known facts and open questions about ultrafilters on omega generating (or not generating) ultrafilters in forcing extensions.
4 November 4 pm UK
Mirna Džamonja
Institut for History and Philosophy of Sciences and Techniques, CNRS & Université Panthéon Sorbonne, Paris and Institute of Mathematics, Czech Academy of Sciences, Prague
On wide Aronszajn trees
Aronszajn trees are a staple of set theory, but there are applications where the requirement of all levels being countable is of no importance. This is the case in set-theoretic model theory, where trees of height and size ω1 but with no uncountable branches play an important role by being clocks of Ehrenfeucht–Fraïssé games that measure similarity of model of size ℵ1. We call such trees wide Aronszajn. In this context one can also compare trees T and T’ by saying that T weakly embeds into T’ if there is a function f that map T into T’ while preserving the strict order <_T. This order translates into the comparison of winning strategies for the isomorphism player, where any winning strategy for T’ translates into a winning strategy for T’. Hence it is natural to ask if there is a largest such tree, or as we would say, a universal tree for the class of wood Aronszajn trees with weak embeddings. It was known that there is no such a tree under CH, but in 1994 Mekler and Väänanen conjectured that there would be under MA(ω1).
In our upcoming JSL paper with Saharon Shelah we prove that this is not the case: under MA(ω1) there is no universal wide Aronszajn tree.
The talk will discuss that paper. The paper is available on the arxiv and on line at JSL in the preproof version doi: 10.1017/jsl.2020.42.
18 November 4 pm UK
Gabriel Goldberg
Harvard University
Even ordinals and the Kunen inconsistency
Abstract. The Burali-Forti paradox suggests that the transfinite cardinals “go on forever,” surpassing any conceivable bound one might try to place on them. The traditional Zermelo-Frankel axioms for set theory fall into a hierarchy of axiomatic systems formulated by reasserting this intuition in increasingly elaborate ways: the large cardinal hierarchy. Or so the story goes. A serious problem for this already naive account of large cardinal set theory is the Kunen inconsistency theorem, which seems to impose an upper bound on the extent of the large cardinal hierarchy itself. If one drops the Axiom of Choice, Kunen’s proof breaks down and a new hierarchy of choiceless large cardinal axioms emerges. These axioms, if consistent, represent a challenge for those “maximalist” foundational stances that take for granted both large cardinal axioms and the Axiom of Choice. This talk concerns some recent advances in our understanding of the weakest of the choiceless large cardinal axioms and the prospect, as yet unrealized, of establishing their consistency and reconciling them with the Axiom of Choice.
2 December 4 pm UK
Kameryn J Williams
University of Hawai’i at Mānoa
The geology of inner mantles
An inner model is a ground if V is a set forcing extension of it. The intersection of the grounds is the mantle, an inner model of ZFC which enjoys many nice properties. Fuchs, Hamkins, and Reitz showed that the mantle is highly malleable. Namely, they showed that every model of set theory is the mantle of a bigger, better universe of sets. This then raises the possibility of iterating the definition of the mantle—the mantle, the mantle of the mantle, and so on, taking intersections at limit stages—to obtain even deeper inner models. Let’s call the inner models in this sequence the inner mantles.
In this talk I will present some results, both positive and negative, about the sequence of inner mantles, answering some questions of Fuchs, Hamkins, and Reitz, results which are analogues of classic results about the sequence of iterated HODs. On the positive side: (Joint with Reitz) Every model of set theory is the eta-th inner mantle of a class forcing extension for any ordinal eta in the model. On the negative side: The sequence of inner mantles may fail to carry through at limit stages. Specifically, it is consistent that the omega-th inner mantle not be a definable class and it is consistent that it be a definable inner model of ¬AC.
Trinity Term 2020
In Trinity term 2020, the seminar is organized by myself and Samuel Adam-Day. In light of the corona virus situation, we will be meeting online via Zoom for the foreseeable future.
For the Zoom access code, contact Samuel Adam-Day me@samadamday.com.
6 May 2020, 4 pm UK
Victoria Gitman, City University of New York
Elementary embeddings and smaller large cardinals
Abstract A common theme in the definitions of larger large cardinals is the existence of elementary embeddings from the universe into an inner model. In contrast, smaller large cardinals, such as weakly compact and Ramsey cardinals, are usually characterized by their combinatorial properties such as existence of large homogeneous sets for colorings. It turns out that many familiar smaller large cardinals have elegant elementary embedding characterizations. The embeddings here are correspondingly ‘small’; they are between transitive set models of set theory, usually the size of the large cardinal in question. The study of these elementary embeddings has led us to isolate certain important properties via which we have defined robust hierarchies of large cardinals below a measurable cardinal. In this talk, I will introduce these types of elementary embeddings and discuss the large cardinal hierarchies that have come out of the analysis of their properties. The more recent results in this area are a joint work with Philipp Schlicht.
20 May 2020, 4 pm
Joel David Hamkins, Oxford
Bi-interpretation of weak set theories
Abstract. Set theory exhibits a truly robust mutual interpretability phenomenon: in any model of one set theory we can define models of diverse other set theories and vice versa. In any model of ZFC, we can define models of ZFC + GCH and also of ZFC + ¬CH and so on in hundreds of cases. And yet, it turns out, in no instance do these mutual interpretations rise to the level of bi-interpretation. Ali Enayat proved that distinct theories extending ZF are never bi-interpretable, and models of ZF are bi-interpretable only when they are isomorphic. So there is no nontrivial bi-interpretation phenomenon in set theory at the level of ZF or above. Nevertheless, for natural weaker set theories, we prove, including ZFC- without power set and Zermelo set theory Z, there are nontrivial instances of bi-interpretation. Specifically, there are well-founded models of ZFC- that are bi-interpretable, but not isomorphic—even $\langle H_{\omega_1},\in\rangle$ and $\langle H_{\omega_2},\in\rangle$ can be bi-interpretable—and there are distinct bi-interpretable theories extending ZFC-. Similarly, using a construction of Mathias, we prove that every model of ZF is bi-interpretable with a model of Zermelo set theory in which the replacement axiom fails. This is joint work with Alfredo Roque Freire.
27 May 2020, 4 pm
Ali Enayat, Gothenberg
Leibnizian and anti-Leibnizian motifs in set theory
Abstract. Leibniz’s principle of identity of indiscernibles at first sight appears completely unrelated to set theory, but Mycielski (1995) formulated a set-theoretic axiom nowadays referred to as LM (for Leibniz-Mycielski) which captures the spirit of Leibniz’s dictum in the following sense: LM holds in a model M of ZF iff M is elementarily equivalent to a model M* in which there is no pair of indiscernibles. LM was further investigated in a 2004 paper of mine, which includes a proof that LM is equivalent to the global form of the Kinna-Wagner selection principle in set theory. On the other hand, one can formulate a strong negation of Leibniz’s principle by first adding a unary predicate I(x) to the usual language of set theory, and then augmenting ZF with a scheme that ensures that I(x) describes a proper class of indiscernibles, thus giving rise to an extension ZFI of ZF that I showed (2005) to be intimately related to Mahlo cardinals of finite order. In this talk I will give an expository account of the above and related results that attest to a lively interaction between set theory and Leibniz’s principle of identity of indiscernibles.
17 June 2020, 4 pm
Corey Bacal Switzer, City University of New York
Some Set Theory of Kaufmann Models
Abstract.
A Kaufmann model is an $\omega_1$-like, recursively saturated, rather classless model of PA. Such models were shown to exist by Kaufmann under the assumption that $\diamondsuit$ holds, and in ZFC by Shelah via an absoluteness argument involving strong logics. They are important in the theory of models of arithmetic notably because they show that many classic results about countable, recursively saturated models of arithmetic cannot be extended to uncountable models. They are also a particularly interesting example of set theoretic incompactness at $\omega_1$, similar to an Aronszajn tree.
In this talk we’ll look at several set theoretic issues relating to this class of models motivated by the seemingly naïve question of whether or not such models can be killed by forcing without collapsing $\omega_1$. Surprisingly the answer to this question turns out to be independent: under $\mathsf{MA}_{\aleph_1}$ no $\omega_1$-preserving forcing can destroy Kaufmann-ness whereas under $\diamondsuit$ there is a Kaufmann model $M$ and a Souslin tree $S$ so that forcing with $S$ adds a satisfaction class to $M$ (thus killing rather classlessness). The techniques involved in these proofs also yield another surprising side of Kaufmann models: it is independent of ZFC whether the class of Kaufmann models can be axiomatized in the logic $L_{\omega_1, \omega}(Q)$ where $Q$ is the quantifier “there exists uncountably many”. This is the logic used in Shelah’s aforementioned result, hence the interest in this level of expressive power.
The seminar talks appear in the compilation of math seminars at https://mathseminars.org/seminar/oxford-set-theory.